If our awareness of the outside world comes from our senses, our reactions to the outside world are controlled by our reflexes.
When we think of reflexes, we typically think of an unexpected reaction to an outside stimulus. We might duck in response to a loud sound, dodge if we see something coming towards us, retract if we touch something hot, or pull away if we stand on something sharp.
All these ‘reflex’ reactions occur when muscles contract in response to an obvious external stimulus.
The reality is, outside forces are stimulating our muscles every time we move, run, jump, walk, throw, catch, get out of bed, or hold our head up, and our reflexes govern our reactions to those forces. Reflexes are ultimately responsible for the action of our muscles and, so, govern the way we move.
Reflexes are, by definition, actions that occur before the brain is aware of what is happening. Reaction to a stimulus is processed in the spinal cord, bypassing brain control. Naturally, if movement results, the brain will become aware of such movement and can regulate it somewhat, but the brain cannot control it.
Reflexes usually have five (and always four) separate parts:
- A Receptor
- A sensory nerve that carries the message from the sensor to the spinal cord
- An interneuron—a relay nerve, as discussed in the previous chapter (Some reflexes bypass the interneuron; others go via three or four interneurons.)
- A motor nerve that carries the message from the spinal cord to the muscle
- A muscle fibre that contracts
One of the best-known reflexes is the knee-jerk reflex. You sit with your leg dangling towards the floor and your leg is tapped just below the kneecap. Hopefully, your foot will shoot out and then relax back to its starting point.
This reflex’s sensors are the muscle spindles located in the quadriceps, or thigh muscle. You will remember that these sensors constantly generate nerve signals.
As the tendon is tapped below the kneecap, a small but sudden stretch is felt by the spindles. The spindles respond by ‘revving’ the engine, i.e., suddenly increasing the frequency of messages to the spinal cord. The muscle spindles’ increased rate of firing relays directly and indirectly to the anterior motor neurons.
The anterior motor neurons now have more sensory input, so their output suddenly increases. The muscle contracts, and, instantly, your foot shoots out.
This reflex does not exist simply for the amusement of doctors and young children. In life, this reflex works to protect our joints from injury.
Imagine a gymnast landing on a mat after a somersault. With her legs bent, she is ready for impact. As her feet touch the mat, her knees bend further. The quadriceps muscle on the front of her thigh starts to stretch. Sensors within the muscle detect the speed and force of the stretch, and fire rapidly. The nerve from the muscle carries the massively increased rate of firing back to the spinal cord, where a direct connection is made to the nerves that control the tone (strength) of the quadriceps. The massive increase in nerve activity is transmitted straight back to the quadriceps, causing it to contract instantly.
The faster the muscle stretches, the faster the response will be. Failure to contract hard enough would cause the gymnast to end her leap sprawled on the ground; too much contraction, and she would be flung straight up in the air again.
The example of the gymnast teaches us that feedback from the stretch of a muscle dynamically alters the muscle strength output in a way that protects our joints and our bodies from injury.
Most of us will never be doing somersaults, but we use those same reflexes every time we walk down stairs, jump off a chair, throw a ball, or bend over to pick up a pen.
Think back to the last time you jumped off a chair, or, if you haven’t done it for a while, try it now. You may have had time to plan the landing, but did your planning really help?
Most of the time, the landing is done on autopilot. Feedback from muscle spindles in your thigh told the motor neurons in your spine exactly how much force to generate so you could land without injury.
Muscles constantly adjust their tone to control our movements.
If you can, squat down on the floor right now. Don’t hold onto anything. Just balance. Notice what is happening to your feet and ankles.
Do you feel the tiny adjusting movements keeping you on balance? These movements are the result of reflexes coming from the muscles themselves. Those same reflexes are operating in every muscle, throughout your body, all day, every day.
Imagine you were walking across rocks and your foot suddenly slipped. The muscles holding your foot would be stretched very rapidly, causing the spindle cells to increase their rate of firing back to the spinal cord. Direct connections to the nerves that contract the muscle are increased, and the muscle suddenly fires, holding the foot, and preventing a sprain of your ankle ligaments.
The reflex that caused your foot to shoot out when the kneecap was tapped, and stopped our gymnast from collapsing on the floor, is actually the same reflex that keeps your ankle safe from sprain or strain while you walk.
Actually, it is unfortunate that the stretch—or myotatic—mechanism was ever called a reflex because most people link reflexes with one-off events, like the knee jerk. But the myotatic mechanism is unlike all other reflexes because it functions twenty-four hours per day, constantly monitoring and maintaining appropriate muscle tone.
When we bend forward to pick up a pen off the ground, we often forget that muscles all the way up our spine are working to hold our weight, that our calf muscles are contracting to push our toes into the ground, and our neck muscles are active to hold our head up. When we pick up a chair or a bag of cement, the sensors in the muscles that move the vertebrae in our spine will have more or less tension placed upon them, and they will vary their output accordingly. As we lift the weight, the amount of tension in the muscles increases, and the muscles increase their power to hold our spine firmly.
These stabilising muscles are vital for our joint function and safety. If the stabilisers don’t work with enough speed or strength, then the joint is likely to be sprained, strained, or damaged. This can happen in one major accident, leading to strain or sprain. Or it can happen over years, causing the wear and tear we know as osteoarthritis.
When you throw a ball, almost the only thing that stops your arm from leaving your body at the end of the throw is the contraction of your shoulder muscles, a contraction that only occurs when the myotatic reflex is activated by the stretch on the muscles.
Understanding the myotatic reflex helps us understand why, despite conventional wisdom, simple stretching is next to useless as a warm- up, warm-down, or injury prevention measure. Every time you stretch, the muscle you are stretching will increase its rate of firing, making that muscle hold even tighter.
Although pre- or post-exercise static stretching has never been shown to prevent injury, nearly all athletes do it religiously, and coaches often insist on it. If you really want to warm up for a sporting activity, or even to mow the lawn, do some push-ups, jump up and down, or go for a short run. Making your muscles work increases the brain input from your muscles, making them stronger, and conditioning your mind and your body for the work ahead.
The stretch, or myotatic, reflex is just one of the reflexes vital to our survival and safety. We also need the withdrawal reflex—the name given to the sequence of events that lifts your foot off a nail, pulls your hand from a hotplate, or causes you to flinch when tickled. It is often called the flexor withdrawal reflex, or the polysynaptic withdrawal reflex, but the simple ‘withdrawal reflex’ is very descriptive because it functions to move us away from danger.
If we stand on a nail, pain sensors in our foot are activated, causing contraction of the hamstrings and calf muscles which lift our foot off the nail. Without such a reflex, we might carry on walking normally and do much more damage. The sensors in our feet respond not just to pain, but also to pressure and light touch. Notice the way we flinch when tickled, and find it difficult to walk with a pebble or other small object in our shoe.
If our gymnast were to land on a nail or sharp stone, feedback from the skin via the flexor withdrawal reflex would activate the hamstrings and cause the quadriceps muscle to be inhibited (switched off). Switching off the very muscles she needs to break her fall would cause her knees to buckle, and she would land in a heap on the floor—but she would not step hard on the sharp object.
When you throw a ball, almost the only thing that stops your arm from leaving your body at the end of the throw is the contraction of your shoulder muscles, a contraction that only occurs when the myotatic reflex is activated by the stretch on the muscles.
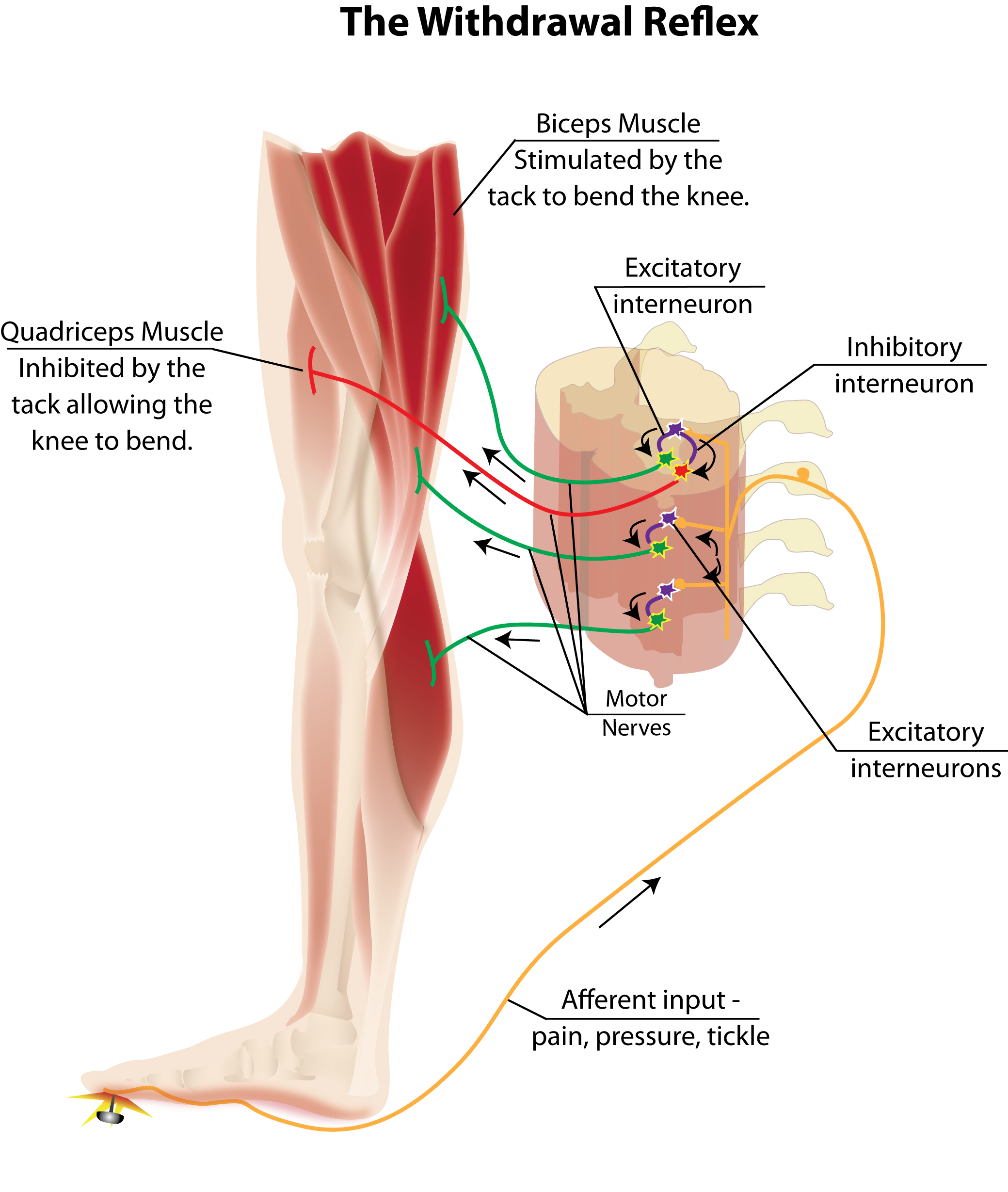
Proprioceptors in our skin detect pain, pressure, light touch, vibration, and temperature. All are linked to relevant muscles by the withdrawal reflex so that, should any of these sensors pick up a sudden change, the part that is threatened will be drawn away rapidly.
You will probably recognise the withdrawal reflex in the feet (standing on a nail) and hands (pulling away after touching a hotplate), but it operates throughout the body. From the agony of biting on a stone to the hysterics of a child being tickled, the withdrawal reflex is always ready to prevent continued irritation, and it does so by altering muscle strength, activating some muscles and inhibiting others.
But the flexor withdrawal reflex does more than just sense danger. The fast connections between sensors in our skin and our muscles help us with movement control.
When we catch a ball, skin sensors will help us gauge its weight. This information is combined with information about the amount of tension on the biceps muscle. That combined information will then be interpreted instantly in the spinal cord so that the right amount of counterforce is generated to allow us to hold the object still, rather than dropping it or throwing it back into the air.
When we think about reflexes, we will naturally think most about the muscles that are actually doing the work.
Since muscles can only pull and never push, they need opposing muscles to pull the joint back to its starting position. Muscles therefore work in pairs, known as agonists and antagonists. An antagonist is simply a muscle (or muscles) that opposes the movement of an agonist. Muscles can shift very quickly from being agonists to antagonists, and back again.
The easiest muscles to use as an example are the biceps and triceps, the muscles that move the elbow. Whenever we bend our elbow, the biceps contracts. As the biceps is contracting, messages are sent to the triceps to temporarily tone it down. The biceps is said to be facilitated, the triceps inhibited.
Sometimes the elbow can straighten with no effort by the triceps (as when we put down a drink), but, if effort is required (e.g., doing a push-up), then the triceps would be facilitated and the biceps inhibited.
Thus, the Law of Reciprocal Inhibition creates muscle partnerships which help control our movements and reactions.
Whenever a motor neuron gets a message to fire, nerves controlling the opposite muscle (or muscles) receive an inhibitory message. In the knee- jerk reflex, facilitation of the quadriceps would accompany inhibition of the hamstrings (the opposite muscle). This ensures the muscle actions don’t cancel each other out.
We are never aware that our muscles are inhibited. We don’t feel anything in our hamstrings when our knee is tapped, even though the hamstrings are temporarily weakened. When we lift our arm, we feel nothing in the muscles that would usually pull the arm down, but they are inhibited none the less.
Much of the control the brain exerts on our movements is actually inhibitory. Without inhibitory input from the brain, our muscles would contract spontaneously, giving the curled-up posture we sometimes see in people who have had a stroke. Thus, smooth movement depends on inhibitory inputs, not just from proprioceptors, but from the brain itself.
Inhibition is not paralysis. If one of the muscles in a pair were completely inhibited, the unopposed muscle action would quickly pull the bones out of position. Our joints would become unstable and dislocated. Partial inhibition allows the opposing muscle to maintain the right amount of muscle tone so that the joints stay in alignment. When the agonist and antagonist muscles work together, the joints are stable but flexible.
The Law of Reciprocal Inhibition plays an important part in the symphony of harmonious and controlled movement.
That ‘law’ operates consistently, throughout the body, during the smallest movement or the most violent. It occurs in the neck, back, hips, legs, abdomen, ankles, feet, and toes—anywhere muscles move bones. Without it, we would find it difficult to move at all. Opposing muscles would compete with each other, and our joints would flop around in an uncoordinated fashion.
The point is that any excitatory input from the stretch (myotatic) reflex or the withdrawal (flexor/polysynaptic) reflex is also inhibitory. Every time an input switches on one muscle, it must be switching off its opposite.
That’s the way our bodies work when they are working properly, and we are avoiding injury.
Simon King